Copper's Role in Radioactive Waste Disposal III - The US Experience
Copper Applications in Health & Environment
US Radioactive Waste Disposal Programs | Copper Makes Its Bid | Yucca Mountain Project | References
In an earlier article, Innovations described the properties that make copper an attractive candidate for containers for the permanent isolation of high-level radioactive waste in an underground repository. A companion article in this month's Innovations tells how Sweden expects to use such copper canisters. This article traces copper's role in the U.S. Department of Energy's (DOE) waste management program. It explains why copper and copper alloys made it to a "short list" of several viable materials and why metals other than copper were ultimately selected.
US Radioactive Waste Disposal Programs 
Radioactive waste ( radwaste for short) comprises all of the leftovers of "nuclear" technology, both military and commercial, during the past 50 years - things as diverse as weapons components, reactor fuel, cancer-fighting medical devices and home smoke detectors. Radwaste ranges from barely radioactive items of soiled clothing to extremely dangerous high-level wastes (HLW), a large portion of which are in the form of commercial spent reactor fuel (CSF) from America's 100-plus nuclear power stations.
America began generating radwastes in the 1940s as byproducts of plutonium production for atomic bombs. Radwaste disposal got little attention in those early days and for a brief period, some wastes were rather casually stored on the grounds of the facilities that produced them.
That practice soon ended. During the Cold War years, large quantities of wastes at nuclear facilities near Hanford, Washington and Savannah River, South Carolina were collected in huge steel-and-concrete tanks to await disposal. No pressing need for a permanent solution to the disposal problem was seen at the time since the wastes presumably weren't going anywhere and it was widely believed that a safe disposal method could eventually be developed. In 1957, the National Academy of Sciences declared confidently that there were "many possible options" for disposing of radwaste safely. True enough, although the scientists ignored the power of public opinion, which would later have an overriding influence on events.
The advent of commercial nuclear power beginning in the early 1960s gave rise to a new form of radwaste, the unreprocessed spent fuel 1 from what have since become more than 100 reactors operating in America's electric generating stations. It is estimated that by 2030, these reactors will have generated between 84,000 and 87,000 metric tons of contained uranium (MTU) as CSF waste in the form of almost
300,000 fuel assembl ies. A typical fuel assembly from a pressurized water reactor (PWR). Each assembly contains approximately 200 zirconium alloy tubes containing the uranium oxide fuel. Copper has been proposed for containers in which to isolate the hundreds of thousands of spent fuel assemblies generated by reactors at electric utilities.
In the 1960s, some of the old tanks at Hanford and Savannah River were found to be leaking. The threat that seepage of radioactive chemicals might threaten public water supplies plus news that radioactive discharges had also occurred at a fuel reprocessing facility in West Valley, New York got the public's attention. A unified program to dispose of radwaste was obviously needed, and in 1970,the Atomic Energy Commission (AEC), now the Nuclear Regulatory Commission (NRC) proposed that the government construct repositories in which to isolate the growing quantity of radioactive detritus.
One experimental repository, excavated into an underground salt bed near Lyons, Kansas had in fact already been built and partially tested. (Salt formations are geologically stable structures and are also impermeable to water intrusion, both desirable features in a waste repository. Some countries plan to isolate their radwaste in salt formations.) The Lyons salt-bed repository initially looked promising, but local citizens' misgivings plus soon-to-follow revelations of radioactive releases at the Rocky Flats weapons facility in neighboring Colorado doomed the project. The NIMBY (Not In My Back Yard!) dictum was born, and cultural/social issues began to assume a stronger voice in the nuclear debate. Also delayed (until quite recently) over public uneasiness was the Waste Isolation Pilot Project (WIPP), another salt-bed repository for defense-type waste near Carlsbad, New Mexico.
As early as 1975, the Energy Research & Development Agency (ERDA) (later the Department of Energy (DOE)) asked the US Geologic Survey (USGS) to identify acceptable underground repository sites, including salt beds and other rock formations. A few years later, the Nuclear Waste Policy Act of 1982 (NWPA) 2 and its 1986 Amendment formally authorized DOE to develop a waste-management plan, including a repository site-selection process. The original plan called for more than three-dozen candidate sites to be screened, of which six would be selected for thorough geologic characterization. What had until then been little more than a collection of pilot-scale investigations coalesced into the multi-billion-dollar enterprise it remains today.
Several national laboratories and numerous government agencies proposed and competitively pursued a number of different waste disposal schemes. An adequate supply of money was available. The NWPA had established a system under which the nuclear power industry would be assessed a 1-mil ($0.001) per kWh fee, passed on to ratepayers, to establish a fund from which all commercial, i.e., other than weapons-related aspects of a repository, including R&D, would be funded. As of fiscal year 1997, that fund had spent $5.5 billion in year-of-expenditure dollars ($6.7 billion in 1998 dollars). A recent review conducted by the Office of Civilian Radioactive Waste Management 3 concluded that the fund was adequate to address the repository program's needs.
A change in government policy in 1987 narrowed the earlier short list of possible repository locations to three sites: one in basalt (a volcanic rock) at the Hanford, Washington nuclear reservation; another in a salt dome in Deaf Smith County, Texas and a third in a type of rock known as welded volcanic tuff under Yucca Mountain, Nevada. Yucca Mountain is a barren ridge located about 100 miles northwest of Las Vegas. It was soon selected as the one site to undergo further characterization, and that site characterization program has since become a massive engineering project that will continue into the next century. 4
As we shall see, the selection of a repository site ultimately determined the type of waste containers that will be buried in it.
Copper Makes Its Bid 
It was during the early-1980s that the Copper Development Association Inc. (CDA) first advanced the concept of copper isolation containers to DOE. CDA's action was prompted by the Swedish nuclear power industry's plan to utilize copper canisters. The Swedish plan is described elsewhere in this issue of Innovations.
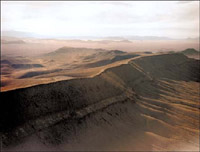
CDA saw copper waste containers as a win-win situation: the environment would be protected by copper's well-known corrosion resistance and U.S. copper miners would have a new market for their product. In the early 1980s, CDA persuaded DOE to include copper and several copper alloys among its candidate canister materials.
CDA presented its proposals to the Yucca Mountain Project Office, then located at the University of California/Lawrence Livermore National Laboratory (LLNL) and to the Westinghouse Electric Company and Pacific Northwest Laboratories, administrators of the Hanford operations. CDA provided scientific and engineering data on copper and its alloys, particularly with respect to the metals' corrosion behavior and long-time stability. CDA also explored fabrication options to demonstrate that the manufacture of copper container was well within today's industrial capabilities.
Materials engineers and corrosion scientists at the Hanford site viewed copper metals quite favorably. Their tests indicated that the local rock and groundwater would not be injurious to copper. Another important item in copper's favor was a DOE-sponsored study 5 of native (metallic) copper deposits in the Keweenaw region of Michigan's Upper Peninsula, a 19 th-Century copper mining district. Copper in the Michigan deposits has remained stable since its formation between 500 and 800 million years ago (some nuggets taken from the mines weighed several tons). Similarities in the geochemical conditions in Michigan and those in the basalt underlying Hanford implied, in the words of the DOE study, "that copper alloys were adequate materials for nuclear waste containers emplaced in a repository located in basalt."
Here was a natural analog that showed how very stable copper would be in a favorable natural environment! The Michigan copper mines were, in fact, proof that a metal could remain uncorroded for a longer time than would be needed in a radioactive waste repository. If copper could last for more than 500 million years given the proper environment, it would certainly be stable enough for a repository that would only have to remain intact for 180,000 years.
Hanford researchers also looked at the effects of radiation. In the presence of air and water, radioactivity can cause the formation of chemical species such as nitric acid, nitrites and ammonia compounds that could attack copper. Studies conducted for the Hanford and Yucca Mountain projects detected some radiation-enhanced corrosion but its implications with regard to copper's performance in an actual repository were inconclusive. Analogous work conducted for the Swedish waste management program suggested that the effect, if any, of this form of corrosion on copper waste containers' integrity would be negligible. 6
Hanford researchers conducted numerous corrosion studies on three copper-base materials proposed by CDA:
- Pure copper, in particular, the oxygen-free variety commonly used in electronic applications. The fact that researchers would be dealing with pure elemental copper makes the prediction of long-term behavior more reliable.
- Aluminum bronze, a corrosion-resistant copper alloy used in the chemical industry and elsewhere. This alloy has good resistance to oxidizing environments.
- Copper-30% nickel, an alloy that is widely used in desalination plants and shipboard steam condensers. Hanford's studies predicted that the near-container environment would be especially benign toward the copper-30% nickel alloy.
Yucca Mountain Project 
CDA continued to provide DOE researchers with information about copper and copper alloys working closely with the team of scientists and corrosion experts at LLNL. Copper and copper alloys were on the list of candidate materials for Yucca Mountain, as were stainless steels, titanium alloys and nickel-base superalloys.
Unlike the Hanford site, the Yucca Mountain repository would be located above the water table. The repository would therefore be quite dry; however, researchers assumed that some water might infiltrate the repository and come in contact with the containers. The water, which would probably resemble local well water, would be relatively non-corrosive to copper in the water's natural state. However, LLNL scientists envisioned a scenario in which water might drip onto containers, evaporate and become concentrated in mineral content, possibly changing its aggressiveness. This could conceivably happen because the U.S. waste containers, unlike those in the Swedish program, would remain above the boiling point for many years after emplacement in the repository.
Corrosion tests were conducted in Yucca Mountain well water at several levels of mineral concentration. Tests were also conducted over the range of temperatures expected in the repository, with and without the presence of radiation and with and without stress. Candidate metals were examined for signs of all the known forms of corrosion, including the particularly insidious form known as stress corrosion cracking. Copper performed well in these tests, but not overwhelmingly better or worse than did other candidate materials.
Consideration of copper continued into the mid-1990s. During this period, an independent team of scientists was appointed by Congress to examine the proposed repository at Yucca Mountain. This panel, known as the Nuclear Waste Technical Review Board (NWTRB), took issue with DOE's plan to use relatively thin-walled (approx. 1 cm, 0.4 in) containers. The scientists favored a more "robust" container similar to that proposed by Swedish engineers.
CDA responded to the NWTRB review by fabricating two thick-walled copper cylinders to simulate the waste containers' dimensions. The first of the two cylinders was made from heavy copper plate, rolled and welded to form a 760-mm (30-in) diameter shell.
The second CDA design took a more innovative approach. It was based on the latest design concepts being advanced at the time by the Swedish waste management program, which called for a robust container having a thick outer shell of pure copper (for corrosion resistance) and an inner shell of structural steel (for strength).
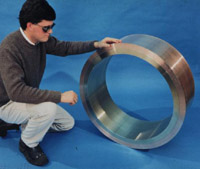
CDA's concept incorporated an outer copper shell with an inner shell of nickel-aluminum bronze, an alloy that is stronger than structural steel but has the added advantage of excellent corrosion resistance. CDA produced the bimetallic container body in one piece by a process known as centrifugal casting.
Centrifugal castings are made by pouring molten metal into a spinning cylindrical mold; centrifugal force holds the metal against the mold wall until it solidifies. Impurities and dross, being lighter than metal, migrate to the inner surface of the solidifying casting, leaving the metal itself relatively clean and defect-free. A large variety of commercial products ranging from cast iron water pipe to propeller hubs for US Navy ships, are made using this process. Wisconsin Centrifugal, Inc., Waukesha, Wisconsin, an industry leader in the use of this casting process, was given the job of developing the prototype container for CDA.
Pure copper was first poured into the spinning mold to form what would become the container's outer shell. Nickel-aluminum bronze was then cast against the nearly solidified copper, bonding the two metals and creating the desired bimetallic structure that can be seen in the photograph, above.
To seal the container, CDA chose a technique known as electron-beam (EB) welding. EB welding bombards the metal to be welded with a high-energy beam of electrons, creating sufficient heat to form a narrow weld joint. The process is very precise (even a two-inch (50-mm) deep joint is scarcely wider than a pencil lead) and because it doesn't cause widespread heating of the waste container.
The novel cast container drew considerable attention at a DOE-sponsored container workshop held in Denver, Colorado in 1991 7 , but government researchers and a new general contractor for the repository program soon thereafter conceived a robust design of their own. It would utilize a very thick outer shell of ordinary steel to serve as a sacrificial "corrosion allowance" material. An inner container made from a nickel-base superalloy would provide corrosion protection after the steel had corroded away.
Despite copper's many advantages, neither CDA nor LLNL researchers could demonstrate unambiguously that copper or copper alloys would last indefinitely in the mildly oxidizing atmosphere expected to prevail in the Yucca Mountain repository. DOE's steel-and-superalloy container got around that problem by allowing the iron to corrode, but simply providing enough of it to last for the time required. One U.S. corrosion scientists referred to this solution as the "big dumb approach".
Today, development of copper radwaste containers continues in Sweden, where it began. Sweden may export its well-developed technology to other countries, as well. Thus, in at least one of the repositories that will be constructed during the coming years, copper will provide a safe and lasting solution to radioactive waste disposal.
References:
1. Much of America's so-called defense high-level wastes resulted from the reprocessing of spent fuel from government reactors. It was originally anticipated that fuel from commercial reactors would also be reprocessed, yielding plutonium for use in other power reactors. A demonstration reprocessing plant was put in operation at West Valley, New York. In part because of concerns over the possible diversion of "civilian" plutonium for military uses, the Carter Administration halted all civilian fuel reprocessing, and the West Valley demonstration project was closed.
2. PC108
3. Office of Civilian Radioactive Waste Management.
4. Yucca Mountain Site Characterization Study.
5. D.P. Crisman & G.K. Jacobs (Michigan Technical University), Native Copper Deposits of the Portage Lake Volcanics, Michigan: Their Implications with Respect to Canister Stability for Nuclear Waste Isolation in the Columbia River Basalts Beneath the Hanford Site, Washington, Rockwell International. DOE Contract DE AC06-77RL01030 (1983).
6. Swedish Corrosion Institute, "Copper as canister material for unreprocessed nuclear waste � evaluation with respect to corrosion," KBS Technical Report 90, No 1978-03-31, Stockholm, 1978.
7. Yucca Mountain Site Characterization Project, Extended Summary Report on Engineered Barrier System Concepts Workshop, June 18-20, 1991, Denver, Colorado. See also: D.T. Peters, K.J.A. Kundig, D.F. Medley and P.A. Anders, Multibarrier Copper-Base Containers for High-Level Waste Disposal, Nuclear Technology, Vol. 104, No. 2, 219(November 1993).
Also in this Issue:
- The Fate of Copper Released from the Vasa Ship Museum
- The Swedish Program for Long Term Isolation of High Level Nuclear Waste in Copper Canisters
- The Copper Motor Rotor: New Technology for High Efficiency Motors
- Shape Memory and Superelastic Alloys
- Copper's Role in Radioactive Waste Disposal III - The US Experience